Mıknatıslar modern toplumun teknolojik dokusunda önemli bir rol oynar, hem günlük eşyalara hem de sofistike makinelere sessizce güç verir. Peki sıcaklığın mıknatısları nasıl etkilediğini merak ediyor musunuz? Bu makale, sıcaklıkların bu kuvvetleri nasıl canlandırdığını veya zayıflattığını inceleyecek, termal enerjinin manyetik malzemeler üzerindeki çeşitli etkilerini ortaya çıkaracak ve dalgalanan sıcaklıklarda manyetik performansı korumak için pratik önlemler önerecektir. Manyetizmanın temellerini ve sıcaklık değişimlerine karşı duyarlılığını anlayarak, bu çok önemli teknolojinin geleceğini şekillendiren eğilimleri ve gelişmeleri tahmin edebiliriz.

Mıknatıslara Giriş
Mıknatıslar, doğanın dört temel kuvvetinden biri olan elektromanyetizmanın bir ifadesi olan manyetizma nedeniyle demir, nikel ve kobalt gibi metalleri çekebilen ilgi çekici varlıklardır. Her malzemenin içinde, atomlar elektron içerir ve mıknatıslarda bulunan elektronlar, daha yaygın olduğu gibi eşit olarak zıt yönlerde değil, ağırlıklı olarak bir yönde yönlendirilir. Bu dengesiz dönüş, kuvvet uygulayan ve çekime veya itmeye neden olan belirgin kuzey ve güney kutupları olan bir manyetik alanla sonuçlanır. Manyetik alan mıknatısın kendi sınırlarının ötesine yayılır ve doğrudan temas olmadan etkileşimlere olanak sağlar.
Manyetizma ve Sıcaklığın Ardındaki Bilim
Sıcaklık ve manyetizma arasındaki etkileşim, atomik hareket ilkeleri tarafından yönlendirilen bir malzeme içindeki atomların hareketi ve hizalanması etrafında döner. Sıcaklık arttıkça, termal enerji bir mıknatıs içindeki atomların daha güçlü titreşmesine neden olur. Bu çalkantı, atomların düzgün bir şekilde hizalanmış manyetik momentlerini bozarak mıknatısın genel manyetik alanını zayıflatabilir ve manyetik gücünde bir düşüşü tetikleyebilir.
Sıcaklık kritik nokta olarak bilinen bir noktaya ulaştığında Curie Sıcaklığı ya da fizikçi Pierre Curie'nin adıyla anılan Nokta'da termal çalkantı o kadar yoğunlaşır ki manyetik hizalamayı bastırarak malzemenin normalde harici bir manyetik alanla hizalanacağı yerde ferromanyetik özelliklerini kaybetmesine neden olur.
Sıcaklık Mıknatısları Etkiler mi?
Evet, sıcaklık mıknatısların manyetizmasını önemli ölçüde etkiler. Hem ısıtma hem de soğutma, bir mıknatısın gücünü, mıknatıslanmaya karşı direncini ve genel performansını çeşitli şekillerde önemli ölçüde değiştirebilir. Bu etkilerin arkasındaki birincil mekanizma, sıcaklığın mıknatıs içindeki atomik ve moleküler yapılar üzerindeki etkisidir.
Sıcaklık Mıknatısları Nasıl Etkiler?
Sıcaklık, mıknatısların manyetik özelliklerini önemli ölçüde etkiler. Sıcaklığın etkisi, sıcaklığın artmasına veya azalmasına bağlı olarak bir mıknatısın çekici kuvvetini güçlendirebilir veya zayıflatabilir. Bir mıknatıs ısıya maruz kaldığında, manyetik alanı zayıflar (Sıcaklık Curie sıcaklığı olarak bilinen belirli bir noktayı aşarsa, mıknatıs manyetik özelliklerini tamamen kaybedebilir). Tersine, bir mıknatıs soğuk sıcaklıklara maruz kaldığında, manyetik özellikleri daha güçlü hale gelir.
Yaygın Mıknatıs Malzemelerinin Curie Sıcaklıkları
Malzeme | Curie Sıcaklığı |
Demir | 770°C |
Nikel | 358°C |
Kobalt | 1121°C |
Neodimyum | 310-400°C |
Yüksek Sıcaklıkların Mıknatıslar Üzerindeki Etkileri
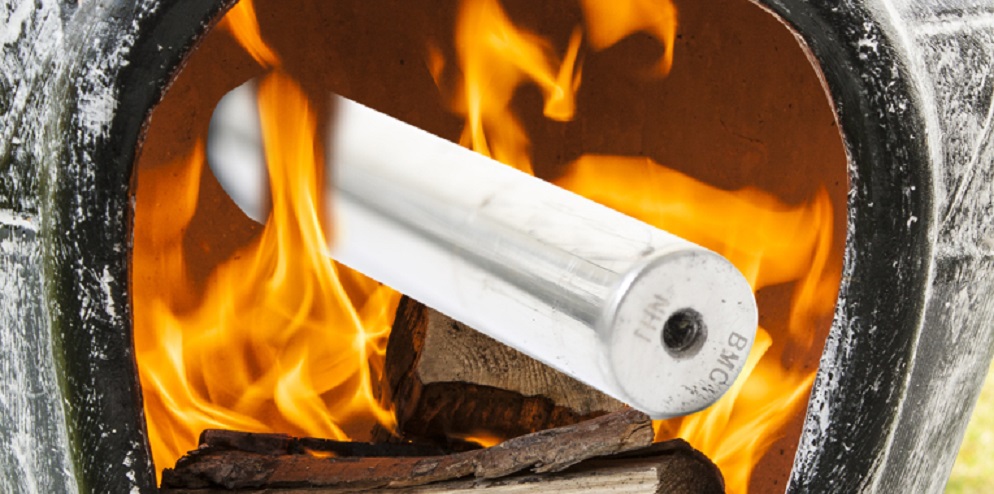
Yüksek sıcaklıklar bir mıknatısın manyetik özelliklerini büyük ölçüde etkileyebilir. Mıknatıslar malzemeye özgü eşik değerlerini aşan sıcaklıklara maruz kaldıklarında, manyetik özelliklerinde bir dizi değişiklik meydana gelebilir.
- Mıknatıslanma Kaybı:
Yüksek sıcaklıklarda, atomlara sağlanan termal enerji titreşim ve hareketin artmasına neden olur. Bu rastgele hareket, malzeme içindeki manyetik alanları (düzgün bir manyetik yönelime sahip bölgeler) hizalayan manyetik kuvvetlerin üstesinden gelebilir. Alanlar yanlış hizalandıkça, malzemenin net manyetik alanı zayıflar ve bu da genel mıknatıslanmasında bir azalmaya yol açabilir. Sıcaklığın Curie noktasının üzerine çıktığını varsayalım. Bu durumda, bu mıknatıslanma kaybı kalıcı hale gelebilir ve malzemeyi artık kararlı bir mıknatıslanmayı sürdüremeyeceği paramanyetik bir duruma dönüştürebilir. - Koersivite Değişimi:
Koersivite, manyetik bir malzemenin manyetikliği giderilmeden harici bir manyetik alana dayanma yeteneğidir. Yüksek sıcaklıklarda, bir mıknatısın zorlayıcılığı tipik olarak azalır. Bunun nedeni, artan atomik hareketin harici bir manyetik alanın manyetik alanları yeniden yönlendirmesini kolaylaştırmasıdır, bu da mıknatısın manyetikliğini gidermek için daha az harici alan gücü gerektiği anlamına gelir. Bu durum, etkinliklerini daha çabuk kaybedebilecekleri için yüksek sıcaklık uygulamalarında kalıcı mıknatıslar için özellikle sorunlu olabilir. - Kalıntılarda Değişim:
Remanence veya kalıntı manyetizasyon, harici bir manyetik alan kaldırıldıktan sonra ferromanyetik bir malzemede kalan manyetizasyondur. Sıcaklıklar yükseldikçe ve Curie noktasına yaklaştıkça, malzemenin güçlü bir manyetik alanı tutma yeteneği azalır. Isıdan kaynaklanan artan enerji, manyetik alanlarının hizalanmasını bozarak malzemenin kalıcı manyetik özelliklerini zayıflatır ve böylece remanansını düşürür. - Enerji Ürünündeki Değişim (BHmax):
Genellikle (BH)max olarak adlandırılan bir mıknatısın enerji ürünü, bir malzemede depolanan manyetik enerjinin yoğunluğunun bir ölçüsüdür. Pratik uygulamalarda bir mıknatısın gücünün önemli bir göstergesidir. Sıcaklık arttıkça enerji ürünü azalır çünkü genel mıknatıslanma ve koersivite azalır. Bu, mıknatısın bir nesneyi kaldırma veya elektrik enerjisini mekanik enerjiye dönüştürme gibi işler yapma kabiliyetinin tehlikeye girdiği anlamına gelir.
Mıknatıs Curie Sıcaklığının altındaki yüksek sıcaklıklardan soğuduğunda, manyetik özelliklerinin bir kısmını geri kazanabilir. Yine de, sıcaklığa maruz kalma bir şekilde yapısını değiştirirse kalıcı kayıplara da sahip olabilir. Yüksek sıcaklıklar içeren uygulamalar için yüksek Curie Sıcaklıklarına sahip malzemelerden yapılmış mıknatısları seçmek ve zaman içinde performansı sürdürmek için sistemi ısıyı etkili bir şekilde yönetecek şekilde tasarlamak çok önemlidir.
Düşük Sıcaklıkların Mıknatıslar Üzerindeki Etkileri
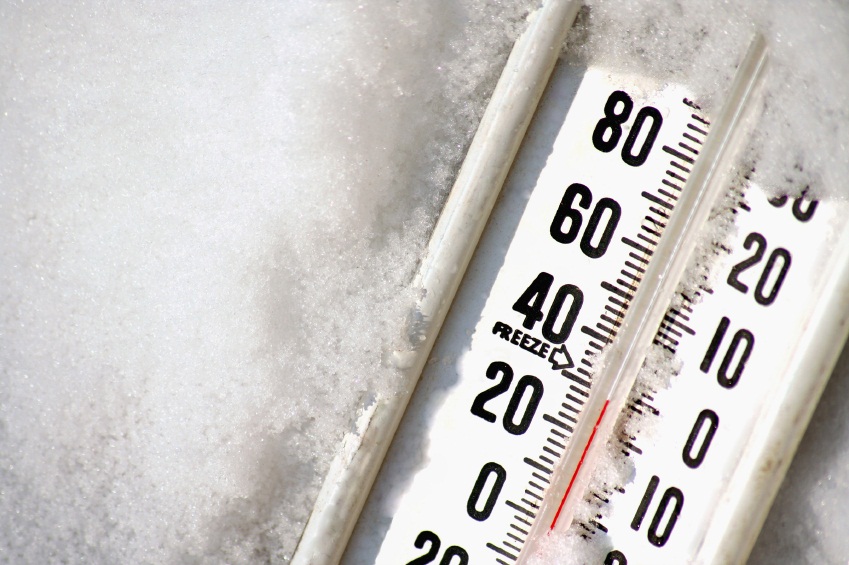
Düşük sıcaklıkların mıknatıslar üzerinde, genellikle yüksek sıcaklıkların etkilerinden oldukça farklı çeşitli etkileri olabilir. Sıcaklık düştükçe, manyetik malzeme içindeki termal titreşimler de azalır. Bu durum manyetik performansta kayda değer değişikliklere yol açabilir:
- Artan Mıknatıslanma:
Termal enerji daha düşük sıcaklıklarda azaldıkça, mıknatıs içindeki manyetik alanlar daha etkili bir şekilde hizalanabilir. Bu da mıknatısın genel mıknatıslanmasını artırır. Rastgele atomik hareketin azalması, manyetik momentlerin hizalanmalarını daha iyi korumalarını sağlar ve böylece malzemenin manyetik özelliklerini geliştirir. - Artan Koersivite:
Koersivite, daha önce de belirtildiği gibi, manyetik bir malzemenin mıknatıslanmasındaki değişikliklere karşı direncidir. Düşük sıcaklıklarda, koersivite genellikle artar çünkü manyetik alanların hizalama etkisi termal enerji tarafından daha az bozulur. Bu, bir mıknatısın manyetik özelliklerini daha iyi koruyacağı ve soğukken mıknatıslanmaya daha etkili bir şekilde direneceği anlamına gelir. - Süperiletkenlik:
Son derece düşük sıcaklıklarda, bazı malzemeler süper iletken bir duruma geçebilir. Süper iletkenler sıfır elektrik direnci özelliğine sahiptir ve enerji kaybı olmadan elektriği iletebilirler. Manyetik özelliklerle ilgili süper iletkenlikle ilgili ilginç bir fenomen Meissner etkisi olarak bilinir. Bir malzeme süper iletken bir duruma geçtiğinde, mükemmel diyamanyetizması nedeniyle tüm manyetik alanları içinden dışarı atacaktır. Bu, bir süper iletkenin manyetik alanlar tarafından nüfuz edilmesine izin vermeyeceği ve mıknatısları etkili bir şekilde iteceği anlamına gelir. Süper iletkenlerin güçlü mıknatıslar yaratmak için kullanılabilmesinin ve manyetik malzemeleri havaya kaldırabilmesinin bir nedeni de budur.
Çoğu malzeme sıcaklık düştükçe mıknatıslanma açısından güçlenirken, bazı sınırlamalar da vardır. Sıcaklıklar mutlak sıfıra yaklaştıkça, bazı malzemeler manyetik yapılarına ve manyetik etkileşimlerinin doğasına bağlı olarak manyetik özelliklerini artırabilecek veya azaltabilecek faz geçişlerine maruz kalabilir.
Pratik Çıkarımlar
Sıcaklık dalgalanmaları, çeşitli günlük ve endüstriyel uygulamalarda kullanılan manyetik malzemelerin performansını ve ömrünü önemli ölçüde etkileyebilir. İşte bu etkileri gösteren bazı örnekler:
- Motorlar ve Jeneratörler:
Sabit mıknatıslar, özellikle kompakt ve verimli çalışma gerektiren tasarımlarda, elektrik motorları ve jeneratörlerin temel bileşenleridir. Bu cihazlar çalışma sırasında genellikle ısı ürettiğinden, mıknatıslar üzerindeki termal etkileri karşılayacak şekilde tasarlanmaları gerekir. Mıknatıslar çok ısınır ve maksimum çalışma sıcaklıklarının üzerindeki sıcaklıklara ulaşırsa, mıknatıslanmalarının bir kısmını kalıcı olarak kaybedebilir, verimliliği azaltabilir ve onarım veya değiştirme gerektirebilir. Güvenilirlik ve performansın kritik öneme sahip olduğu rüzgar türbinleri veya elektrikli araçlar gibi endüstriyel uygulamalarda malzeme ve tasarım seçerken bu hususun göz önünde bulundurulması gerekir. - Manyetik Depolama Ortamı:
Sabit diskler ve diğer manyetik veri depolama cihazları verileri kaydetmek için manyetik malzemeler kullanır. Yüksek sıcaklıklar, verileri depolayan manyetik alanların hizasını kaybetmesine neden olarak verilerin bozulmasına veya kaybolmasına yol açabilir. Bu cihazlarda depolanan verilerin güvenilirliği ve uzun ömürlülüğü için kontrollü bir sıcaklık ortamının korunması şarttır. - Manyetik Levitasyon (Maglev) Trenleri:
Maglev trenleri, treni minimum sürtünme ile kaldırmak ve itmek için güçlü süper iletken mıknatıslar kullanır. Süper iletken durumlarını korumak için, bu mıknatıslarda kullanılan süper iletken malzemelerin genellikle sıvı helyum veya nitrojen ile son derece düşük sıcaklıklarda tutulması gerekir. Sıcaklık kritik sıcaklığın üzerine çıkarsa, süper iletkenlik özellikleri kaybolur ve tren havalanamaz veya verimli bir şekilde hareket edemez. - MRI Makineleri:
MRI makineleri, görüntüleme için gereken manyetik alanları oluşturmak için güçlü süper iletken mıknatıslar kullanır. Bu mıknatıslar, verimli çalışma için süper iletken kalmaları amacıyla kriyojenik sıcaklıklarda tutulur. Herhangi bir sıcaklık artışı süper iletken durumdan çıkışa yol açabilir ve bu da mıknatısı tekrar operasyonel sıcaklıklara soğutmak pahalı ve zaman alıcı olduğundan maliyetli olacaktır. - Parçacık Hızlandırıcılar:
MRI makineleri gibi, Büyük Hadron Çarpıştırıcısı (LHC) gibi parçacık hızlandırıcılarındaki süper iletken elektromıknatısların da verimli çalışabilmesi için çok düşük sıcaklıklarda tutulması gerekir. LHC, mıknatıslarını 1,9 Kelvin'de tutmak için sıvı helyum kullanıyor. Sıcaklıktaki herhangi bir artış süperiletkenliği söndürebilir, bu da potansiyel olarak operasyonel bir kapanmaya yol açabilir ve mıknatısların hasar görmesi riskini doğurabilir.
Endüstriyel uygulamalar için, sıcaklık hususları genellikle belirli mıknatıs türlerinin seçilmesine yol açar. Yüksek sıcaklıklı ortamlar için, samaryum-kobalt gibi daha yüksek Curie sıcaklıklarına sahip malzemelerden yapılmış mıknatıslar, daha düşük Curie sıcaklığına sahip neodim-demir-bor gibi diğer mıknatıslara tercih edilebilir. Geniş bir sıcaklık aralığında manyetik özelliklerdeki değişiklikleri hafifleten malzeme veya tasarım çözümlerini içeren sıcaklık dengeleme teknikleri de kullanılabilir.
Mıknatısları Sıcaklık Etkilerinden Korumak için İpuçları
Mıknatısları sıcaklık dalgalanmalarının olumsuz etkilerinden korumak ve performanslarını sürdürmek için bu ipuçlarını dikkate alın:
Doğru Malzeme Seçimi
Beklenen sıcaklık aralığına uygun manyetik malzemeler seçin. Örneğin, alniko mıknatıslar sıcaklık değişimlerinde en iyi mukavemet kararlılığını sergiler, ancak manyetikliği gidermeye karşı en düşük dirence sahiptir. Neodimyum mıknatıslar genellikle ferrit mıknatıslardan daha yüksek sıcaklıklara dayanabilir ancak daha pahalıdır. Samaryum-kobalt mıknatıslar sıcaklık değişimlerine karşı daha yüksek direnç sunar ve yüksek sıcaklıklı ortamlar için en iyi seçim olabilir.
Yaygın Kalıcı Mıknatısların Manyetik Özellikleri
Malzeme | Maksimum Çalışma Sıcaklığı | Manyetik Alan Gücü | Maliyet |
Alnico | 600°C | 0.5-1.3 T | Düşük |
Ferrit | 180°C | <0.4 T | Çok Düşük |
Samaryum Kobalt | 350°C | 1,1 T'ye kadar | Yüksek |
Neodimyum Demir Bor | 230°C | 1,4 T'ye kadar | Orta düzeyde |
Termal Yönetim
Sabit bir sıcaklığı korumak için soğutma sistemleri uygulayın. Isıyı mıknatıstan uzağa dağıtmak için yüksek sıcaklıklı ortamlarda ısı alıcıları, fanlar veya sıvı soğutma sistemleri kullanmayı düşünün. Termal izolasyon, mıknatısların düşük sıcaklıklar için optimum çalışma sıcaklıklarında tutulmasına yardımcı olabilir.
Manyetik Devre Tasarımı
Düşük sıcaklık katsayılı manyetik özelliklere sahip malzemeler kullanarak sıcaklık etkilerini en aza indiren manyetik devreler tasarlayın. Bazı tasarımlar ısıyı manyetik malzemeden uzağa da yönlendirebilir.
Çevresel Kontrol
Mıknatısın etrafındaki sıcaklığı belirli bir aralıkta tutmak için klima veya ısıtma ile çevresel koşulları koruyun. Mıknatısı dış sıcaklık değişimlerinden izole etmek için muhafazalar kullanılabilir.
Sıcaklık Telafisi
Sıcaklık dengeleme malzemeleri kullanın. Bazı mıknatıslar, çeşitli sıcaklıklarda manyetik çıktıyı dengelemeye yardımcı olabilecek karşıt sıcaklık genleşme katsayılarına sahip malzemelerle eşleştirilebilir.
Yüksek Sıcaklığa Maruz Kalmayı Sınırlandırın
Mıknatısları motorlar veya ısıtma elemanları gibi ısı kaynaklarının yakınına yerleştirmekten kaçının. Uygulama yüksek sıcaklıklar içeriyorsa, görev döngülerinin soğutma sürelerine izin verdiğinden emin olun.
İzolasyon
Mıknatısları aşırı sıcaklıklardan korumak için ısı yalıtımı uygulayın. Bu, özellikle dış mekan uygulamalarında veya sıcaklıkların büyük ölçüde değiştiği ortamlardaki mıknatıslar için önemli olabilir.
İzleme
Mıknatısın sıcaklığını gerçek zamanlı olarak izlemek için sıcaklık sensörleri uygulayın. Otomatik sistemler sizi sıcaklık anormalliklerine karşı uyarabilir ve koruyucu soğutma veya ısıtma mekanizmalarını tetikleyebilir.
Bakım ve Test
Kritik uygulamalardaki mıknatısların manyetik özelliklerini düzenli olarak test ederek hala beklenen parametreler dahilinde performans gösterdiklerinden emin olun. Termal stres nedeniyle bozulan mıknatısları değiştirmek veya yeniden mıknatıslamak için bakım programları oluşturun.
Üretici Kılavuzları
Sıcaklık aralıkları ve kullanım için üreticinin spesifikasyonlarını takip edin. Üreticiler genellikle malzemelerinin sıcaklık katsayılarına ilişkin yararlı veriler sağlar ve optimum çalışma koşulları önerir.
Her uygulamanın özel gereksinimleri ve kısıtlamaları olacaktır, bu nedenle mıknatısları sıcaklık etkilerinden korumak için yaklaşımı uyarlamak, uzun ömürlülüğü ve güvenilirliği açısından önemlidir. Son kullanım senaryosunu göz önünde bulundurun ve maliyetli yeniden tasarımları veya arızaları önlemek için sıcaklık etkilerini göz önünde bulundurarak tasarım yapın.
Son Araştırmalar ve Gelişmeler
Manyetik malzemelerdeki son araştırma ve gelişmeler, sıcaklık değişimlerine karşı hassasiyeti azaltan malzeme ve teknolojilere odaklanmaktadır. İşte bu alandaki bazı gelişmelere kısa bir genel bakış:
- Sıcaklık etkileri üzerine genel tartışma: Küçük ve ince mıknatıslar genellikle hacim olarak daha büyük mıknatıslara göre yükselen sıcaklıklara karşı daha hassastır. Daha fazla ayrıntı için şu adresi ziyaret edebilirsiniz Magnet Expert'in teknik tavsiye sayfası.
- Sıcaklığın SMC Malzemeleri Üzerindeki Etkisi: Yumuşak Manyetik Kompozit malzemelerin manyetik ve enerjik davranışları üzerinde çalışma sıcaklığının etkisi üzerine bir çalışma yapılmıştır. Deneysel sonuçlar, şu adreste bulunabilecek bir makalede rapor edilmiştir MDPI.
- Malzemelerin sıcaklık kararlılığı: Bazı malzemelerin sıcaklığın etkilerine karşı diğerlerinden daha duyarlı olduğu belirtilmektedir. Alnico mıknatıslar, daha düşük zorlayıcılığa sahip olmalarına rağmen, sıcaklık değişiklikleri altında en iyi mukavemet stabilitesine sahip oldukları belirtilmektedir. Bu konuda daha fazla bilgiye şu adresten erişilebilir US Magnetix.
- Temperature’s impact on magnet performance: An article discusses how high temperatures can partially or entirely demagnetize magnets, whereas lower temperatures can improve magnetic field strength. This is detailed in GME Magnet’s knowledge base.
These resources offer a glimpse into the varying susceptibility of magnetic materials to temperature changes and some ongoing research addressing these challenges.
Sonuç Olarak
In summary, when it comes to protecting magnets from temperature effects and maintaining their performance, several key points have been discussed:
- Selection of the Right Material: The choice of magnetic materials should align with the expected temperature range of the application, with options like samarium-cobalt for high-temperature conditions or ferrite for a balance of cost and thermal resistance.
- Termal Yönetim: Cooling systems, insulation, and environmental controls can help maintain stable temperature conditions, reducing the risk of thermal demagnetization.
- Manyetik Devre Tasarımı: Creating designs that can help mitigate the effects of temperature fluctuations and maintain magnetic performance across different temperatures.
- Monitoring and Testing: Using sensors to monitor temperatures and regularly testing magnetic properties ensures that magnets remain within their performance specifications.
- Research Trends: Ongoing research into Soft Magnetic Composite materials, high-temperature permanent magnets, and advanced cooling techniques represent the future cutting edge of magnet technology.
The future of magnet technology seems poised to build upon these foundation points. It will likely be characterized by innovations in materials science – creating new magnetic materials or composites with improved thermal stability, advanced manufacturing techniques that enhance magnetic performance at various temperature ranges, and smarter thermal management systems integrated within applications using magnets.
As demands for high-performance magnetics with robust temperature tolerance grow—especially for use in advanced electronics, renewable energy technologies, and electric transportation—the industry will continue to seek solutions that push the boundaries of how we understand and utilize magnets’ properties. The continuous research and material advancements in the magnetic field are quite promising for engineering applications requiring reliability in diverse, temperature-extreme environments.